Defining LDES
LDES is the short form for the term Long Duration Energy Storage. In simplest terms, it can be described as the solution, system, or technology used to store some form of energy in stable form for utilization on demand later. LDES solutions are seen as the essential enablers to accelerate the replacement of fossil-fuel-based energy generation, especially in meeting peak demands. Energy is stored from renewable generation sources during excess generation periods and released or utilized during peak-demand periods when renewable energy may not be readily available. This system provided not only flexibility and security but will also become a major key in the carbon neutralization of European grids (EASE, 2018).
There is no global standard definition for LDES. The term is typically used when referring to storage devices that can store electrical or heat energy for a rated duration of time. LDES term is also used when referring to systems that provide stability or peak-shaving capacity to electric grids. Another, rather fashionable context, to which this term is frequently linked, is when talking about battery storage systems. No matter the context, the two common factors are always the duration in units of time and capacity in units of joules. The time duration of the energy stored referred to may vary from as low as 15 minutes up to 720 hours, meaning several months or seasonal storage. For purely benchmarking and communication consensus reasons a duration of 4 hours to 10 hours is commonly used by regulatory authorities, academic researchers, and general media publications (Denholm et al., 2021; EASE, 2018). In addition, various other factors are important in the design and definition of LDES. These factors can be broadly classified into two categories: a) technological and b) project considerations, presented in Table 1, adapted from (Howitt, 2018) below.
Technology factors | Project considerations |
---|---|
Rated Power: For example, a 20MWh battery could produce 10MW for 2 hours or 40 MW for 30 minutes | Cost of system and project: Includes initial investment as well as operating costs. |
Capacity: For example, a grid-connected peak shaving battery system having a capacity of 0.25 to 2 hours at the full-rated output | Operation lifetime: This will define the ultimate return on investment and the feasibility of the project. |
Response time: how fast the energy system can be available and synchronized with the grid. | Environmental consideration: Rare-earth materials mining or effects of construction of stored hydropower plant. |
Duty cycles: The charging/discharging profile associated with the energy storage system | Energy Supply and demand: Is there enough energy to charge the system (surplus) and is there enough demand for discharged energy (beyond the peak demand)? |
Other technological factors such as overall efficiency and losses, need for ancillary systems as well as total life span. | Other factors such as social acceptance, availability of components, power purchase agreements, and geo-political scenarios. |
Figure 1, based on research from the European Association for Storage of Energy, shows the classification of various energy storage technologies. Each technology or system of energy storage has an essential role to play in its own category, and some example projects are presented in this article. The type of technology adopted will ultimately depend on the application and combination of the various factors as presented in Table 1 above.
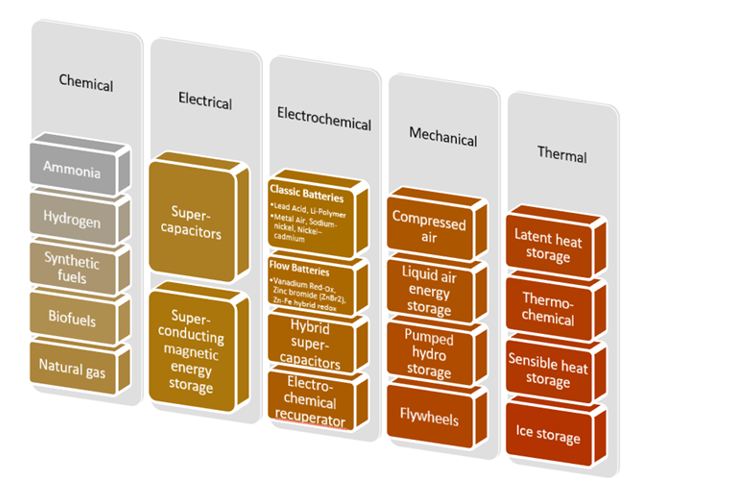
Electrochemical energy storage
The category of up to 4 hours to 6 hours of energy storage is well suited for a variety of battery chemistries, including lithium-ion, nickel-based, sodium-based, and lead acid. Research suggests that by the year 2030, Lithium-ion batteries will dominate the battery energy storage markets globally. The increased global awareness of emission-free transportation will even further enhance this development (Beuse et al., 2020; Shan et al., 2022).
One of the largest BESS in Europe is in Olkiluoto, in Finland and will go into operation during 2022-23. This combined venture between Hitachi Energy and Finnish nuclear power company, TVO boasts an output capacity of up to 90 MW. The main function of this system is to provide energy backup to the grid, in case of production disruption in the Olkiluoto 3 nuclear power plant. More information and pictures of this project are available on TVO project website.
An excellent example of emissions reduction in transportation by using BESS is the ultramodern Aurora Bothnia Wasa line, one of the world’s most environmentally friendly roll-on/roll-off passenger ferries. This 150-meter-long car and passenger ferry have onboard four liquified natural gas reciprocating engines to charge the 2.2 MW Lithium-ion battery system provided by Leclanché SA energy storage solutions. The battery pack is utilized for port entry/departure, peak shaving, hotel load, and boost power, greatly reducing the load on main engines and thus emissions and noise. More information on the ferry is from the ferry operator’s website, Kvarken link.
Pumped hydro energy storage
This type of energy storage is classified under the mechanical forms of energy storage and is one of the most conventional forms of energy storage. The system design is composed of reservoirs at different elevations or potential differences. Energy is stored by pumping water to the higher potential reservoir and subsequently, energy is released under influence of gravity to flow water over turbines that are further connected to electrical generators. The challenge in Pumped hydro plant construction is always the topographical factors. One intelligent example to utilize human-made structures to advantage of energy storage is the Pyhäjärvi pumped hydro energy storage power station in Pyhäsalmi, Finland. This project claims the highest hydraulic head in the world in the category of underground energy storage plants. The key behind this project is the re-utilization of abandoned mine structures where the mine shafts go as deep as 1400 meters. The plant, when operational will have a capacity of 530 MWh, and delivers 75 MW electrical power over 7 hours period. More interesting graphics and technical details from the project website at PYHÄJÄRVEN CALLIO.
Thermal hot water storage
One of the most important uses of energy in northern Europe is heating. In the year 2019, the production of district heat in Finland amounted to 38.1 TWh. In comparison, in the same year (2019), the production of electricity in Finland amounted to 66.0 terawatt hours (TWh). Heat is an important form of energy storage for arctic climate countries(Statistics Finland, 2020).
Heat storage is also one of the most common modes of energy storage. The heat can be generated from solar energy combined with heat pumps or other energy sources like wind-to-electricity, gas, heating oil, or biofuels. It’s the storage part that needs design and consideration. One excellent example of a large-scale thermal energy storage facility is the EPV-Vaskiluoto, plant in Vaasa, Finland. In the year 2020, this was the largest operating LDES-thermal facility in Finland. This project utilizes underground taverns to store up to 210,000 m3 of heated water. Heat is generated by burning biomass from forest industry. The plant’s storage capacity is around 7 – 9 GWh and its discharge capacity is 100 MW. More information and detailed technical specification are available at project website at EPV-Vaskiluodon voima.
Conclusion
Energy storage has many interesting and important applications throughout the energy system. LDES solutions will be an important factor in future carbon-neutral energy ecosystems. The range of applications that energy storage devices can provide is ever-changing, due to the continued development of new energy storage technologies, but also to the changing needs of energy systems for flexibility. It is expected that the list of hosting applications will continue to grow over the next few years.