Defining Power-Sector Transformation
The power-sector transformation is a complex process that involves changes in the energy infrastructure, regulations, and economic incentives. It requires the adoption of new technologies and business models, as well as the development of market designs and innovative solutions to meet the growing energy demand of low carbon society (see Figure 1).
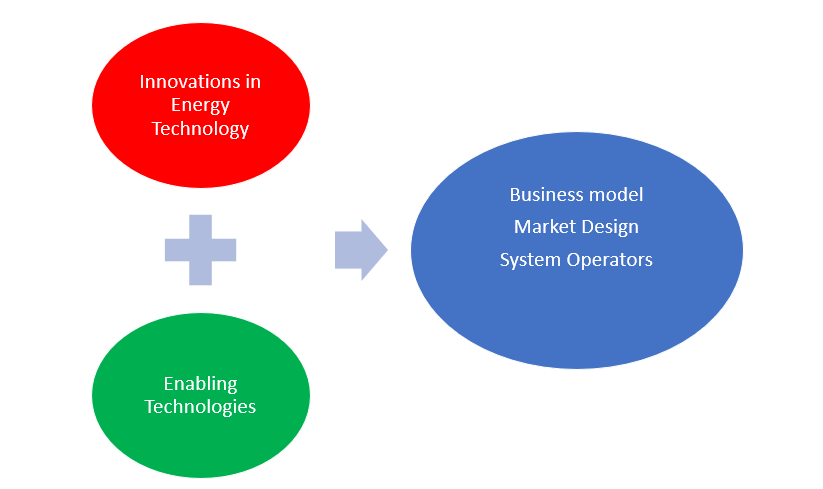
Innovations in energy technologies
The current innovations in energy technologies include energy storage, distributed energy and micro grids. Energy storage systems can store excess energy, allowing it to be used when needed. Distributed energy resources such as rooftop solar and microgrids can help increase the reliability and resilience of the power grid. Drones are employed for predictive maintenance in wind energy farms.
The energy technology innovations that are emerging are having impacts on market design, business models and systems operation (IRENA, 2019). To understand the trends in adopting these innovations, researchers (e.g., Huenteler et al., 2016; Malhotra and Schmidt, 2020) have defined energy innovations in terms of complexity and customization (See Figure 2). Customization is determined by three factors: user preferences, regulatory contexts, and physical environments. Energy technologies with low design complexity consists of small number of components and modular interfaces between components. In contrast, design intensive technologies are characterized by a high number of components and multiple interactions between them. Thus, their design, manufacturing and assembly involve high inputs of technical and management skills for product design and supply chain management. High complex technologies consist of even higher number of components and interactions among them. The considerable number of different actors and the various sectors involved in the design, manufacturing and assembly of the components, sub-systems and the final product often necessitates a more project-based form of organization (Hobday, 2000; Malhotra and Schmidt, 2020). Standardized technologies require no design variation. Such technologies are highly flexible and can be used in different environments with little or no adaptation (e.g., solar photovoltaic). In comparison, certain technologies might need to be customized to a certain extent to fit their use environment (e.g., biomass power plants). When the product design and production processes represent a mix of standardized, mass-produced products and highly customized products, they are known as mass customized technologies (Huenteler et al., 2016; Hobday, 2000). This distinction is extremely critical since it is related to market structure, type of research cooperation, location specific, nature of investments, and the type of supply chain required to ensure that the deployment of these technologies match the goals of climate change. Understanding these differences can also impact the type of policies and investments required to accelerate the goals of low carbon society.
Huenteler et al. 2016 found that solar PV technology followed the life-cycle pattern of mass-produced goods. In contrast, wind turbine technology follows the life cycle of complex products and systems. For example, in Figure 2, Type 1 low-carbon energy technologies have low design complexity and low customization such as solar photovoltaic (solar PV) modules and light-emitting diode (LEDs) and the cost of these technologies has reduced significantly in past years due to economies of scale in mass manufacturing (Huenteler et al., 2016). Type 2 technologies, such as rooftop solar PV and wind turbines, have standardized core modules for different environments. On the other hand, Type 3 innovations, which are highly complex such as combined cycle gas turbine (CCGT) plants, carbon capture and storage, bioenergy with carbon capture and storage (BECCS) and hydrogen production are developing at a slower pace. Adapting energy technologies to their environment is a key requirement for low carbon society. Customized technologies such as geothermal power plants, pumped hydro storage, and biomass power plants in Type 3 typology have relatively lower global adoption rates.
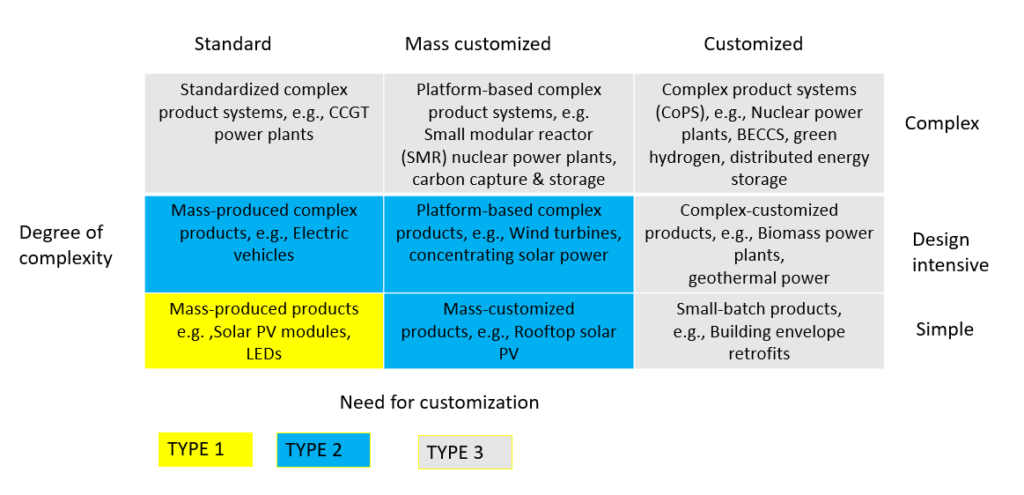
Enabling Technology for Power-Sector Transformation
The power-sector transformation is being enabled by a range of technologies, including information technology. The Internet of Things (IoT) is used to assisting to monitor and control energy systems. Artificial intelligence is being employed to optimize energy systems and improve energy efficiency. Advanced metering infrastructure can help improve energy efficiency by monitoring energy use and allowing consumers to make informed decisions about their energy consumption. Smart grids are networks of connected devices that can monitor and control the flow of electricity. These technologies can help reduce energy costs and increase the reliability and affordability of energy services.
Business Models for Power-Sector Transformation
The power-sector transformation is creating new business models. These business models can help increase the efficiency, reliability, and affordability of energy services. One example of a new business model is energy-as-a-service, which allows customers to pay for energy services on a subscription basis. This model can help reduce the cost of energy services and increase the reliability and affordability of energy services. Another example of a new business model is peer-to-peer energy trading, which allows customers to buy and sell energy directly from each other. This model can help reduce energy costs and increase the efficiency of the power grid. Finally, distributed energy resources such as rooftop solar and microgrids are creating new business models such as energy cooperatives and energy communities. These models can help increase the reliability and resilience of the power grid and reduce energy costs.
Market Design for Power-Sector Transformation
The power-sector transformation creates new market designs. Market designs such as energy auctions and demand response programs can help increase the efficiency and affordability of energy services. Energy auctions are market mechanisms that allow customers to bid for energy services. This can help reduce energy costs and increase the efficiency of the power grid. Demand response programs are market mechanisms that allow customers to provide energy services when needed.
System Operators for Power Sector Transformation
The increase in the share of renewable energy in the energy mix is changing the operation of power systems. The distribution and decentralisation of the system requires new operation practices such as improved cooperation between distribution and transmission system operators. The intermittent nature of wind and solar energy requires advanced forecasting methods and tools. For example, new models could improve the accuracy and detail of forecasting, by using cloud-based computing, improved mathematical models, and machine learning (to produce forecast results in 15 minutes instead of an hour). Better forecasting data decreases uncertainty for system operators, resulting in better integration of renewable energy. Other operational practices to increase flexibility are emerging, such as the use of pumped hydro storage. With renewable energy sources such as wind located from demand centres grid congestion occurs for limited periods of time (IRENA, 2019).
Challenges Faced in Power-Sector Transformation
Innovation in the power-sector transformation is not without its challenges. These challenges include regulatory barriers, the cost of innovative technologies, the complexity of the energy system and the need for customization of certain energy technologies (Malhotra and Schmidt, 2020). Regulatory barriers can limit the adoption of innovative technologies and business models. The cost of innovative technologies can be a barrier to adoption. And the complexity of the energy system can make it difficult to implement innovative energy technologies and business models at a rate that matches the goals of achieving low carbon society. Finally, the lack of public awareness and acceptance of the power-sector transformation can be a barrier to adoption.
Conclusion
The power-sector transformation is a complex process that involves changes in the energy infrastructure, regulations, social behaviours and economic incentives. It requires the adoption of innovative technologies and business models, as well as the development of market designs and innovative solutions. The power-sector transformation creates benefits, including reducing carbon emissions, improving air quality, and reducing energy costs. It can also create new jobs and economic opportunities and help move the world towards a low-carbon future. However, there are also challenges that need to be addressed, including regulatory barriers, the cost of innovative technologies, the complexity of the energy system, and developing appropriate supply chain models. From the education perspective, it is vital to follow these new innovations and trends in the power sector so that we can develop the competences and skills of our students to meet the challenges of a low carbon society.