Energy system design is a key factor in the sustainability of our environment. It is becoming increasingly important to understand and apply the principles of modelling energy system design to meet the demands of today’s energy needs. This article provides an overview of the key concepts and techniques that can be used to design energy systems for low carbon society. It explains the basics of energy system modelling, the components that make up a model, the different types of models approach, and the advantages and challenges associated with modelling energy systems. By understanding the principles of modelling energy system design, we can make informed decisions about the appropriate way to design efficient, sustainable energy systems for low carbon society (LCS). Energy system analysis has been defined as a system approach bringing together multiple perspectives and disciplines to solve energy planning issues viewed as complex problems. Energy system analysis is useful when dealing with interactions between energy, economy and society, as well as when considering competition between the energy sectors for investment needs (Nakata et al., 2010).
What is a carbon low society?
A low carbon society is one that relies on low carbon technology for its sustenance and growth instead of fossil fuels. A low carbon society uses renewable energy sources such as wind, solar, and hydroelectric power to produce electricity. The future of low carbon society would be dominated by 4Ds (decarbonization, democratization, decentralization and digitalisation).
Decarbonization
Decarbonising” electricity involves reducing the amount of carbon released into the atmosphere when electricity is generated. A low carbon society is one that is also concerned with environmental sustainability by using green technologies that are more resource-efficient and help reduce harmful emissions. A low carbon society is also one that is concerned with reducing CO2 emissions through technological advancements, behavioural changes and energy-efficient technologies.
Democratization
The democratization of energy is the process of making clean and affordable energy accessible and available to everyone. In other words, it’s breaking down the barriers that limit access to energy and shifting energy to the hands of individuals, and consumers. For example, rooftop PV systems, can help meet the electricity needs of buildings where they are installed as well as neighbouring buildings and communities. The new energy future will also be shifting economic power from large corporations into the hands of individuals and consumers. The democratization of energy is not a specific technology. It is a concept that is expected to be driven by a variety of different technologies that aim to make energy affordable and accessible to everyone.
Decentralisation
Decentralisation refers to a change towards the production of electricity from small-scale power sources distributed throughout an electricity grid, often co-located with loads (i.e., sources of electricity demand). Webb et al. (2020) suggests that the increase of decentralisation is due to the reducing cost of renewable energy technologies, such as solar PV and wind; the availability and decreasing cost of new technologies for storing electricity (e.g., batteries); and the increasing feasibility of incorporating energy efficiency and demand response into grid and distribution system operations.
Digitalisation
Digitalisation is also driving investment in renewable energy sources, like wind and solar, and playing an important role in the transition to a low-carbon future. Digitalisation refers to the use of advanced communication technologies to facilitate system management, including such things as time-of-use electricity meters and other “smart grid” technologies that allow real-time adjustments and more efficient operation of the local grid, community energy resources, and buildings (IRENA, 2019). Digitalisation can enable producers and consumers to know in real time, the status of energy systems, coordinate local distribution grids, as well as a real-time balance of supply and demand across larger grid areas. In addition, the growing importance of digitalisation is also due to developments in decentralisation and electrification. Decentralisation creates large numbers of new energy producers, such as rooftop PV. Electrification of transport and heat means large quantities of new loads, such as electric vehicles (EVs), and heat pumps. All these creates new assets on the supply side (due to decentralisation) and demand side (due to electrification) have an impact on power systems, making monitoring, management and control crucial for the success of the energy transformation in a low carbon society (IRENA, 2019).
What is energy system modelling?
Energy systems are made of components that be categorised as either inputs or outputs. The inputs are the fuels or feedstocks that are required to produce the desired energy outputs, such as electricity from a power plant, natural gas from a pipeline, or a synthetic feedstock produced from a chemical plant. These may include electricity to power appliances, heat for climate control, or movement of freight through a transportation system. Energy system modelling helps us to understand how these components interact with each other and how to design effective energy systems that meet the needs of society such as a low carbon society. Energy system modelling is a process that requires a combination of engineering skills and knowledge of the behaviour of the system. Energy system modelling can be done at the level of a single device or component, or the entire system, and can range in complexity from simple spreadsheet models to large and complex computer codes.
The power system organisational structures that are dominant today were developed in era of conventional electricity generation, that is for large, centralised, dispatchable and mostly fossil fuel-based generation with high variable costs. The transition to LCS requires the need to design new energy modelling system to reflect the 4Ds. The concept of systems approach for energy systems design for the LCS, is presented in Figure 1. This concept presents a general framework on how the design of energy system is linked to the 4Ds.
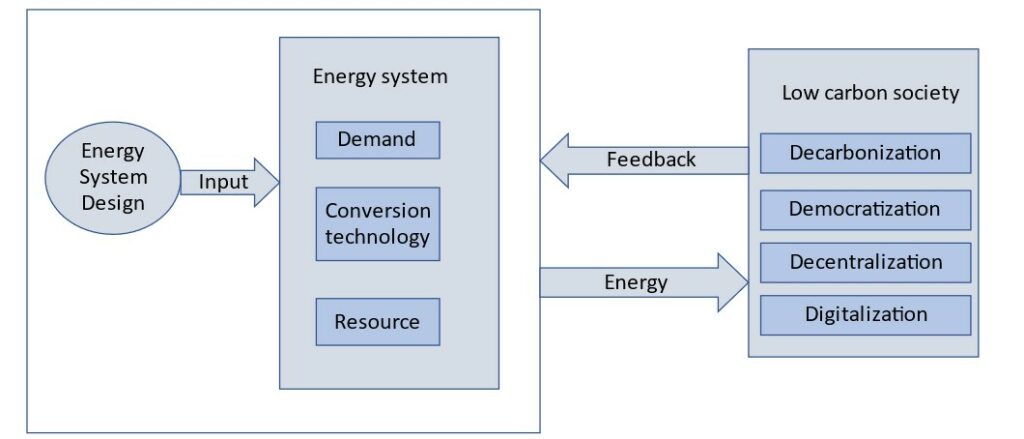
Different Types of Energy System Models
Energy system modelling encompasses a wide range of different modelling types that can be used to analyse different aspects of a system. The revised modelling approach for energy systems for LCS are based on analytical approach, purposes of the analysis, methodology, mathematical approach, geographical coverage, time horizon, data requirements and people (see Table 1). Previous researchers (e.g., Nakata et al. 2010) do not include and consider the role that people, and communities, can play. People are the focus of this paradigm shift, working together as instruments of change. Consumers can act as active stakeholders engaged in the development of new social practices related to energy use. Adopting these perspectives allows decision makers to formulate new ways to engage the public on climate and energy issues, ultimately encouraging society to adopt more low-carbon behaviours (NCC, 2016).
Approach | Category |
---|---|
Purpose | forecasting |
Analytical | top down and bottom up |
Methodology | econometrics, optimization, simulation, multi criteria |
Mathematical | linear programming, mixed integer |
Geographical | global, regional, national, communities, local |
Time horizon | daily, weekly, monthly, yearly |
Data requirements | quantitative, qualitative, aggregated, and disaggregated. |
People | ethnographic studies |
Modelling can help us to understand the potential of distributed energy resources (DERs) and the impact that they will have on the energy system. A model can help us to understand how the various DERs will interact with the grid, and their potential impact on the system’s reliability, stability, and economics. Modelling can also help us to determine the optimal location for a DER, and to assess which DERs would be most beneficial to include in a system. For example, modelling can determine the optimal location for a solar panel, and whether that solar panel would be more beneficial powering an electric vehicle charging station or plugging directly into the grid. Models can also help us to test different scenarios, enabling us to easily identify potential barriers to the adoption of DERs and potential solutions. This can help us to accurately forecast how much a system will cost, how much power it will generate, and how it will operate. This can help us to determine whether a particular system is feasible or if there are any barriers to its implementation.
While modelling can help us to understand the potential impacts of DERs and optimise energy systems, it also introduces several uncertainties that can make predicting the future more difficult. Modelling works by building a virtual representation of the system in question, and then using this model to predict future outcomes based on certain inputs. There are many factors, however, that can influence the accuracy of these inputs and, therefore, the accuracy of the model. Modelling energy systems will always involve some level of uncertainty, and there are many different factors that can introduce inaccuracies into the model. These include inaccurate descriptions of the system, inaccurate inputs, and inaccurate modelling assumptions.
Conclusion
The trend for low carbon societies is to “decentralize, decarbonize, democratize, and digitalize”. These trends, also known as the “four Ds”, are caused by the need to reduce electricity costs, change aging infrastructure, enhance resilience and reliability, reduce CO2 emissions to mitigate climate change, and provide reliable electricity to areas lacking electrical infrastructure. It is important to recognise the common challenges of 4D transitions and the energy systems needed to address them. Energy system modelling is an important tool in the design of sustainable energy systems for LCS, although there are many uncertainties that can affect the accuracy of its predictions. Future energy system design for LCS should take into account the role people play in the transformation of energy systems. It can help us to understand how different technologies will interact with one another, and the grid and can help us to accurately forecast the costs of a system. Modelling energy systems is a complex and time-consuming process, but the rewards are significant.